Sam Santhosh, who spearheaded India's genomic revolution through the incubation and launch of many companies that harness the power of sequencing and bioinformatics, holds a positive outlook on India's advancement in the bioeconomy. In an exclusive interview, he sheds light on India's potential as well as the areas where it needs to improve in genomics and drug research. Excerpts:
What are the advancements in bioeconomy in India?
The bioeconomy encompasses a wide range of industries, primarily in the life sciences, but also bio-manufacturing. Numerous advancements are occurring, with the genomics revolution being particularly influential. Genome sequencing, in particular, serves as a cornerstone for this revolution. The breakthrough began in 2008-2009 with the emergence of new technologies enabling faster and more affordable sequencing.
It is a remarkable transformation where sequencing projects that used to take months can now be completed in a few days. This change has revolutionised the field of biology, as it has become digitised. The key element in the bioeconomy is biology itself, and, fortunately, the source code of life is universal across all living organisms. Consequently, the technologies for genomic sequencing and the necessary tools for analysis are applicable to all living organisms. Many advancements have emerged, including the retrieval of ancient DNA from bones that are thousands of years old. These breakthroughs have significantly enhanced our understanding of evolution and the shared genes across all species. To comprehend evolution, it is crucial not only from a scientific perspective but also for gaining insights into diseases and potential improvements in life.
We are significantly behind the US and China in spite of the advancements. The US holds the leadership position, but China has made remarkable strides in the past 20 years, particularly in pushing the bioeconomy applying genomics. They have invested substantial funds and effort in its development, making them a close second to the US. China has also produced a considerable number of publications and made significant advancements in real-life applications, surpassing the progress of both the US and India. In a democratic environment, certain limitations exist in pushing some of these advancements, whereas China, being a more dictatorial regime, can effectively implement new initiatives across the country. In terms of research and applications, China is currently ahead of India, but India has shown promise primarily in applications, albeit lagging in pure research. It is reasonable to expect India to catch up in about 20 years.
What major innovations can we anticipate in genomic research in this decade? How will these advancements change how we deal with human health and diseases?
The genomic revolution progressed through three phases. The first phase involved reading the genetic code through sequencing technology. The genetic code consists of four letters, A, G, C, and T, representing the four chemicals that make up DNA. All living organisms are based on these four letters, although their order and length vary. For instance, the human genome consists of approximately three billion letters in each of our cells, while other organisms may have larger or smaller genomes. The size of the genome does not necessarily indicate the complexity or development of an organism, but it provides insights into the evolution and specific traits exhibited by the organism.
Genes play a crucial role in determining an organism's traits, and genes from one organism can often function properly in another organism. However, transferring genes between organisms requires extensive work to ensure they work correctly within the new host. From a gene's perspective, it operates like a part of a software program and is compatible across organisms. The understanding of these concepts was made possible through gene sequencing.
As the cost of sequencing decreased and the speed and power of sequencing technology increased, vast amounts of data became accessible. This data offered valuable insights into the causes of diseases and why certain individuals are more prone to them, and facilitated the development of new targets for medicines. The Covid-19 pandemic highlighted the significance of sequencing as it enabled the rapid identification of the virus and played a vital role in developing vaccines within a remarkably short period. These advancements and discoveries characterise the first phase of the genomics revolution.
With the vast amount of genomic data available, we can now begin to manipulate it through editing. This process is similar to how language develops, starting with learning words and gradually developing the ability to converse and write. Similarly, we can now edit the genetic code. Editing plant genomes has progressed rapidly, though they are more complex yet present fewer ethical concerns. In the US, for instance, more than 60 per cent of food consists of genetically modified plants. This technology allows for improved crop yields, reduced pesticide and fertiliser use, increased shelf life, and enhanced nutrition. Unfortunately, many countries, including parts of Europe and India, have expressed fears about genetically modified organisms, despite these fears being unfounded. However, the Indian government has relaxed regulations now and categorised genetically modified food into three categories, allowing for more genetically modified food to emerge in the second wave of the genomic
revolution.
Genomic editing is also being explored for human applications. The first human trial for a genome editing medicine is currently underway in the US by Verve Therapeutics, with successful completion of the first phase of clinical trials and plans for Phase 2. They aim to find a solution for high cholesterol through gene editing. High cholesterol levels contribute to heart disease, and while there are existing medications, they often require lifelong use and have side effects. The researchers are focusing on a specific gene called PCSK9 that, when altered by changing a single letter, prevents cholesterol increase. Previous evidence shows that altering this gene does not cause other problems since many individuals naturally lack the gene. The goal is to administer a single injection to modify this specific letter in the gene through a process known as base-pair editing.
When genome editing gained popularity, CRISPR technology received significant attention. This approach involves cutting the desired region of the genome and introducing desired changes. While it is easy to use, it can have unintended consequences due to the cutting and reattachment process. In contrast, base-pair editing provides greater control as it targets specific letters for modification, minimising potential side effects.
Gene and cell therapies have gained significant attention in the last decade. However, the current processes are expensive and inaccessible to the average Indian.
They are very expensive. Even in the US, they cost like a million dollars per patient. So even there very few people can afford it. For instance, CAR T-cell therapy involves modifying T-cells, which are our immune fighters, to recognise and target cancer cells. Typically, cancer cells evade our immune system, allowing them to grow unchecked. By extracting T-cells from the body, modifying them to specifically recognise cancer cells, and reintroducing them, the immune cells can effectively eliminate the cancer. However, this process is time-consuming and costly. Lowering the cost of CAR T-cell therapy poses significant challenges.
Researchers are exploring various approaches to make allogenic CAR T-cell therapies more feasible and affordable. Allogenic therapies involve using T cells from a few donors and modifying them to be suitable for anyone with specific cancer and gene mutation. By leveraging sequencing data, the specific line of modified T-cells can be quickly administered to patients. If mass manufacturing can be achieved, the cost of these therapies could be significantly reduced. However, the challenge lies in overcoming the complexities of the immune system, as each person's immune response is unique. Despite the challenges, efforts are being made in this direction to reduce costs.
Another avenue of exploration is the use of different immune-related cells in the body, such as NK cells (natural killer cells). Researchers are investigating these alternative cell types for potential therapeutic applications. Additionally, there are ongoing advancements in other technologies related to cell therapy and gene therapy. It's worth noting that the current CAR T-cell therapies primarily target liquid cancers, as solid tumours present greater challenges due to their heterogeneous nature. Unlike liquid cancers, solid cancers like lung cancer can have multiple mutations and different types of cancer cells within them. Developing CAR T-cell therapies that effectively address solid cancers remains an ongoing area of research.
Numerous companies are actively working on various diseases, including rare diseases that carry a significant burden in India. Many of these rare diseases are caused by single gene mutations, often involving just a single letter in the genetic code. The use of base pair editing technology, as mentioned earlier, holds great potential for addressing these diseases by making precise fixes. Over the next 10-20 years, we can expect significant advancements in gene editing that will contribute to the treatment of many diseases.
The third phase of the genomic revolution will involve synthetic biology, which encompasses the creation of new genomes and the manipulation of genes to manufacture a wide range of products, from fertilisers to cosmetics to medicine. This will entail using cell lines, yeast, and other microbes to produce desired substances, scaling up production in large fermenters. Various forms of sugar, biomass, and even waste materials can serve as inputs, enabling environmentally friendly and sustainable production processes. The bioeconomy will bring forth a plethora of products that are safe for the environment and free from toxic by-products. The potential impact of this development is immense, and it will far exceed the current target of a $300 billion market.
CUT, PASTE, CURE
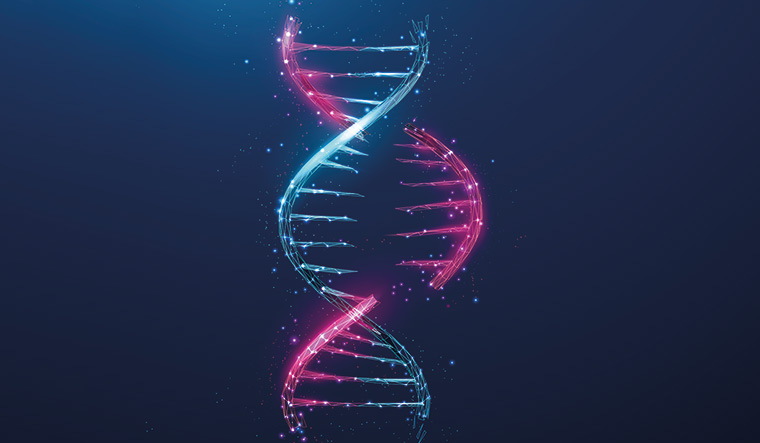
Genetic code
The genetic code consists of four letters, A, G, C, and T, representing the four chemicals that make up DNA (adenine, guanine, cytosine and thymine). The two strands of DNA are held together by bonds between these bases.
All living organisms are based on these four letters, but their order and length vary. The human genome, for instance, consists of around 3.3 billion letters in each of our cells, while a dog genome has around 2.8 billion.
Gene editing
It is now possible to edit the genetic code. Editing plant genomes has progressed rapidly. In the US, more than 60 per cent of food consists of genetically modified plants.
The first human trial for a genome editing medicine is under way in the US, looking for a solution for high cholesterol. The researchers are focusing on a specific gene called PCSK9 that, when altered by changing a single letter, prevents cholesterol increase. The goal is to administer a single injection to modify this specific letter in the gene through base-pair editing.
CRISPR gene editing vs base-pair editing
Clustered Regularly Interspaced Short Palindromic Repeats gene editing involves cutting the desired region of the genome and introducing desired changes. It can have unintended consequences due to the cutting and reattachment process. In contrast, base-pair editing provides greater control as it targets specific letters for modification, minimising potential side effects.